top of page
In recent years, more and more attention has been paid to the presence of toxic metals in the environment - and the link between toxic metals and cancer, neurotoxicity, immunotoxicity, cardiotoxicity, reproductive toxicity, teratogenesis, and genotoxicity. Mechanisms of toxicity include inhibition of enzyme activity and protein synthesis, alterations in nucleic acid function, and changes in cell membrane permeability.
Metals exist in several valence states and form a variety of organic and inorganic compounds. While many are essential to living systems (and serve a variety of cellular, physiological, and structural functions), others are toxic - even at very low levels. Their accumulation in the environment is of concern because they are not biodegradable.
All metals can cause disease through excess. Toxic effects are dependent upon the amount ingested, entry rate, tissue distribution, concentration achieved, and excretion rate.
Acute metal toxicity due to occupational/industrial exposure can be readily diagnosed by means of patient history, overt symptoms and certain toxicological tests. The subtler effects of chronic, low-level exposures are associated with rather nondescript symptoms, however, and overt expressions of physiological aberrations are often not realized until later in life - this is particularly true for the neurotoxic effects associated with the sulfhydryl-reactive metals such as arsenic (As), cadmium (Cd), lead (Pb), mercury (Hg), and palladium (Pd).
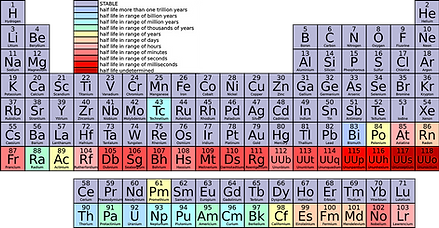
Click Here
Toxic metals are not just a modern-day issue; humans have succeeded in poisoning themselves throughout history. Lead usage dates back to 2000 BC; arsenic was used to decorate Egyptian tombs; and historians contend the fall of the Roman Empire was hastened by chronic lead poisoning (the ruling classes drank water from lead pipes and wine from lead/alloy goblets).
Generally, metals produce their biological toxicity by forming complexes or "ligands" with organic compounds. These modified biological molecules lose their ability to function properly; the affected cells either malfunction or die. The most common groups involved in ligand formation are oxygen, sulfur, and nitrogen. When metals bind to these groups, they affect protein structure or render important enzyme systems inactive. Patients with a metal-metabolism disorder can be especially at risk since they tend to accumulate, rather than eliminate, these toxins.
Toxic metals tie up the cysteine in our food and, when the protective endogenous thiols (metallothionine and glutathione) are saturated, they trap proteins, peptides, enzymes, lipoic acid, and other thiols critical to the proper functioning of our bodies. This causes nutritional deficiencies of critical amino acids, enzymes, and minerals necessary for numerous metabolic functions.
The sulfhydryl-reactive metals (As, Cd, Pb, Hg, and Pd) have three major properties that mechanistically explain how they elicit a majority of their toxic effects. First, they are transition metals that promote the formation of hydrogen peroxide and enhance the subsequent iron- and copper-induced production of lipid peroxides and the highly reactive hydroxyl radical. Lipid peroxides alter membrane structure and are highly disruptive of mitochondrial function.
The pro-oxidant properties of the metals are exacerbated by their inhibitory effects on antioxidant processes. Hg and Cd have high affinities for glutathione (GSH), which is the primary intracellular antioxidant and conjugating agent. Importantly, a single atom of Cd or Hg can bind to, and cause the irreversible excretion of, up to two GSH tripeptides. The metal-GSH conjugation process is desirable in that it results in the excretion of the toxic metal into the bile. However, it can deplete the cell of GSH and thus decrease antioxidant capacity. Lead-induced depletion of intracellular GSH and increased levels of malondialdehyde in the brain and liver have been demonstrated in animal models. It has also been demonstrated that Hg not only directly removes GSH from the cell, but also inhibits the activities of two key enzymes involved in GSH metabolism: GSH synthetase and GSH reductase. Hg also inhibits the activities of the free radical quenching enzymes catalase, superoxide dismutase, and perhaps GSH peroxidase. The inhibition of GSH peroxidase has been attributed to the formation of a mercury-selenide complex. Selenium is an integral component of GSH peroxidase.
Certain “transition metals” like nickel, cadmium, mercury and palladium are carcinogenic to humans. However, certain mechanisms of their carcinogenic activity remain obscure. One possible mechanism would involve metal-mediated promutagenic oxidative damage to DNA and nuclear proteins. Another would include adverse effects on the fidelity of DNA replication and repair processes, as well as derangement of gene expression and cell signaling, through interactions with essential metals and/or induction of conformational changes in biomolecules.
As with all metals, these “transition metals” are both ductile and malleable, and conduct electricity and heat. The interesting thing about transition metals is that their valence electrons, or the electrons they use to combine with other elements, are present in more than one shell. This is why they often exhibit several common oxidation states. And it is this unique ability that allows them to inhibit antioxidative processes and thereby create reactive oxygen toxic species (ROTS). In general, the transition metals promote excessive production of lipid peroxides and hydroxyl radicals that inhibit antioxidative enzymes. These also inhibit a plethora of other enzyme families by virtue of their high affinity for free sulfhydryl groups (e.g. Creatinine kinase, Na/K ATPase). The metals deplete intracellular glutathione (GSH), and inhibit enzymes involved in the synthesis and metabolism of the important antioxidant and metal-conjugating peptide. Though this site introduces only 5 of the 38 transition metals (cadmium, copper, mercury, nickel, and palladium) there are other potentially toxic transition metals noteworthy of mention: iron, cobalt, gold, manganese, chromium, and tungsten.
The elements classified as "other metals" are ductile and malleable; they are not the same as the transition elements. These elements, unlike the transition elements, do not exhibit variable oxidation states, and their valence electrons are only present in their outer shell. All of these elements are solid, have a relatively high density, and are opaque. They have oxidation numbers of +3, ±4, and -3. Of these, we have links to aluminum and lead on this site; other noteworthy potentially toxic metals in this category are tin, bismuth and thallium.
Biological Interactions of Toxic Metals
That's not all ...
In addition to promoting lipid peroxidation, depleting GSH and inhibiting antioxidative processes, the sulfhydryl-reactive metals disrupt the structure and function of numerous important proteins through direct binding to free sulfhydryl groups. Intact sulfhydryl groups are critical for the biological activities of virtually all proteins, including Na/K ATPase. Metal-induced inhibition of Na/K ATPase can result in astrocytic swelling and destruction (astrocytes are the primary cells responsible for the homeostatic regulation of synaptic pH, Na/K and glutamate, and metal sequestration in the CNS). Recent studies clearly illustrate how destructive the interaction between Hg and sulfhydryl groups can be. Hg inhibits the polymerization of tubulin, causes depolymerization of existing microtubules, and in animal studies results in brain lesions that closely resemble those found in patients with Alzheimer's.
the sulfhydryl-reactive metals
The body makes important adaptive changes in response to exposure to sulfhydryl-reactive metals. Studies in rats illustrate the importance of Glutathione (GSH) metabolism in the presence of Hg exposure. Short and long-term exposure to methyl-mercury (MeHg) in drinking water resulted in a two- to three-fold up-regulation of mRNA encoding for g-glutamylcysteine synthetase, which is the rate-limiting enzyme in GSH synthesis. Concomitantly, there was a similar magnitude of increase in the steady state levels of GSH, and the activities of GSH reductase and GSH peroxidase. These data illustrate a protective, adaptive response to Hg exposure in renal epithelial cells. Neurons do not appear to have such adaptive capacity, which may partially explain why Hg is relatively more neurotoxic then nephrotoxic.
A second adaptive and protective response to toxic metal exposure is induction of metallothionein synthesis. Metallothioneins are a fascinating group of low molecular weight, intracellular proteins that serve as a storage depot for copper and zinc, and "scavenge" sulfhydryl-reactive metals that enter the cell. Metallothioneins across species are rich in cysteine (~30%) and have higher affinities for the transition metals mercury and cadmium than for zinc. Therefore, as Hg and Cd bind to metallothionein, and are restricted from entering the mitochondria, zinc is released. The free, ionized zinc, which would be toxic if permitted to accumulate, binds to a metal regulatory element on the promoter region of the metallothionein gene and "turns on" the synthesis of metallothionein. Such induction of metallothionein provides increased binding capacity for both toxic metals (protective) and zinc (functional). The displacement of zinc in the presence of toxic metal burden may explain in part why increased levels of zinc are so commonly seen in the scalp hair of patients exhibiting significant levels of toxic metals Hg, Cd, Cu, Pb.
The importance of metallothionein in the protection against toxic metals is evident. Mammalian cell lines with the greatest number of copies of the metallothionein gene, and the highest levels of metallothionein survived exposure to Cd in culture media. MT-null mice, genetically engineered to have inactivated metallothionein genes, died within three days of exposure to Cd in drinking water, while control (normal) mice did not exhibit any signs of Cd toxicity. Rat pups exposed to Hg vapor in utero were born with higher levels of metallothionein mRNA and metallothionein levels in astrocytes. Metallothionein levels are also induced in primary astrocyte cultures by CdCl2 and MeHg. The induction of metallothionein in astrocytes is very important in protecting the CNS since neurons cannot up-regulate GSH or metallothionein synthesis in response to metal exposure.
Adaptive Responses to Metal Toxicity
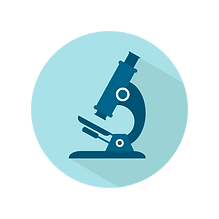
Chris Shade, PhD on Chemical & Heavy Metal Detoxification
Chelation and Detoxification
While metals remain one of the most hazardous environmental toxicants known to man, researchers dedicated to investigating metal toxicity have achieved considerable success in analyzing risk assessments in humans, identifying the diagnostic markers and revealing the underlying pathways.
Chelation has been the mainstay therapy in metal poisoning and related disorders. The term chelate was first used by Sir Gilbert T. Morgan and H.D.K. Drew in 1920. They suggested the term for the caliper-like groups which function as two associating units and fasten to a central atom to produce heterocyclic rings. The Greek word chelate means "claw" and the process of ring formation was termed chelation. In molecular terminology, the larger the number of ring closures to a metal atom, the more stable the compound. This phenomenon is called the “chelate effect” and is generally attributed to an increase in the thermodynamic quantity called entropy that accompanies chelation. The stability of a chelate is also related to the number of atoms in the chelate ring.
Chelating agents offer a wide range of sequestrants to control metal ions in aqueous systems. By forming stable water-soluble complexes with multivalent metal ions, chelating agents prevent undesired interaction by blocking normal reactivity of metal ions.
For these compounds to be of therapeutic relevance they must
-
be water soluble;
-
be resistant to biotransformation;
-
cross through physiological barriers into compartments where a toxic metal ion is accumulated (the mesenchyme, the cells);
-
form a stable complex with the metal that is non-toxic;
-
be easily excreted from the site of deposition and body and not recycled into other tissue;
-
exhibit low affinity for essential metals (Ca, Zn, Cu, Mn, Mg, Se, Fe).
Commonly known chelating agents include calcium disodium ethylenediaminetetraacetic acid (CaNa2EDTA), 2,3- dimercaptopropanol also known as British Anti-Lewisite (BAL), D-Penicillamine, meso-2, 3-dimercaptosuccinic acid (DMSA), 2, 3-dimercaptopropanesulfonic acid (DMPS), polyaminocarboxylic acids diethylenetriaminepentaacetic acid (DTPA), cyclohexanediaminetetraacetic acid (CDTA), hydroxycarboxylic acid sodium catechol 3, 5-disulfonate (Tiron), dithiocarbamates (DDC), desferrioxamine (DFO) and deferiprone (L1).
CaNa2EDTA is probably one of the most commonly used chelating agents (mainly known for application against lead poisoning). CaNa2EDTA is administered intravenously as dextrose or saline infusion due to poor gastric absorption.
The most commonly prescribed oral chelator today is DMSA (its chemical acronym), also known as Chemet. This is a prescription drug available in an oral formulation only; it is approved for use in the treatment of lead poisoning in pediatric patients with blood lead levels >45 μg/dL. While generally safe, DMSA has been associated with mild elevations in hepatic transaminases and allergic reactions. DMSA is also used in the treatment of mercury and arsenic poisoning although these are not FDA-approved indications.
Most chelating agents used today have side effects, some potentially serious - caused primarily by the release of toxic metals into the bloodstream and into the kidneys. Most of the “safer” chelating agents (CaNa2EDTA, DMSA) show partial efficacy in case of chronic metal exposure due to their inability to cross physiological barriers. CaNa2EDTA cannot pass through cellular membranes and therefore its use is restricted in removing metal ions from their complexes in the extracellular fluid. Similarly, conventionally used DMSA shares the limitation of extracellular distribution.
This is why chronic poisoning (the slow accumulation of metal inside the cells) becomes difficult to address, and why chelation therapy has been both challenging and long debated. Although conventionally known chelating agents do show therapeutic efficacy, more specific and safer compounds are needed. Moreover, supplementing conventional chelation therapy with essential minerals and metals (zinc, selenium, sulfur, manganese) has also been shown to improve metal detoxification outcomes.
Oxidative stress may be considered one of the prime contributing mechanisms in metal toxicity and thus provides a strong rationale for including antioxidants during chelation therapy. Combinational therapies with antioxidants such as lipoic acid, vitamin C, N-acetyl cysteine, and glutathione have shown considerable promise in improving clinical recoveries in animal models.
Several botanicals and naturally occurring substances (algae, cilantro, clay, shilajit, gossypin), also are effective compounds for chelation support or substitution.

Chelate is the Greek word for "claw".
Click the links below for information* on the following ten common and highly toxic metals: aluminum (Al), antimony (Sb), arsenic (As), beryllium (Be), cadmium (Cd), copper (Cu), lead (Pb), mercury (Hg), nickel (Ni) and palladium (Pd).
*Specific sources of exposure, biochemical interactions, body tissues in which the metal tends to be deposited, health effects, and common chelating, complexing and conjugating detoxification agents.
Ten Toxic Elements
Click for more information on each individual element.
bottom of page